A Janus emitter for passive heat release from enclosures
by Thamarasee Jeewandara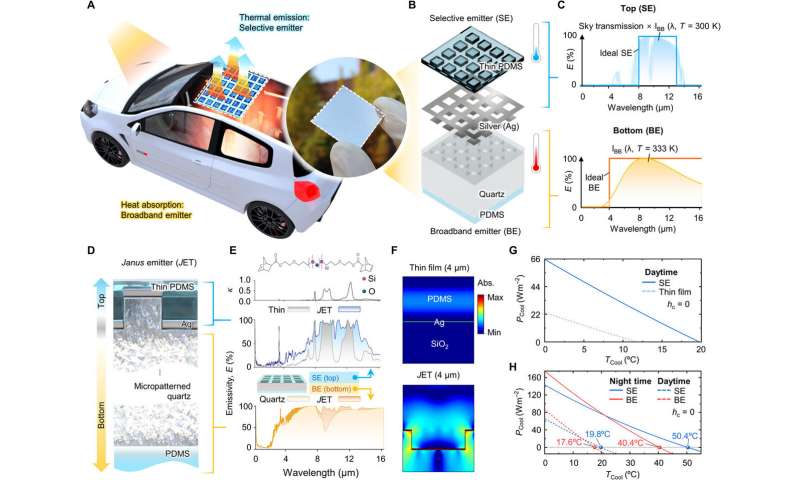
It is presently challenging to efficiently cool enclosed spaces such as stationary automobiles that trap heat via the greenhouse effect. In a new report in Science Advances, Se-Yeon Heo and a team of scientists in materials science, engineering and nanoarchitectonics in Japan and the Republic of Korea, presented a Janus emitter (JET) for surface cooling. They used a silver (Ag)-polydimethylsiloxane (PDMS) layer on a micropatterned quartz substrate and the material allowed them to cool the space even when the JET was attached within an enclosure. As a result, the JET (Janus emitter) could passively mitigate the greenhouse effect in enclosures and offer surface cooling performance comparable to conventional radiative coolers.
Cooling technologies
Present-day cooling technologies depend on vapor compression and fluid-cooled systems, but they consume approximately 10 percent of the global energy, while accelerating the depletion of fossil fuels. Between 1990 and 2018, the amount of carbon dioxide (CO2) emissions from space cooling have more than tripled to reach 1130 million tons, alongside escalating issues of ozone depletion and air pollution. The Earth can cool itself via radiative cooling a passive thermal management strategy to emit unwanted heat to outer space without energy consumption, and passive radiative coolers have shown sub-ambient cooling when attached to exterior materials such as the roof or even human skin to draw heat through convection or conduction in the daytime. However, such strategies can be ineffective during extreme heat accumulation in stationary vehicles, where extremely high temperatures can develop under the greenhouse effect due to transparent windows that allow solar radiation to enter, while being opaque to the exiting long-wave thermal radiation. In this work, Heo et al. proposed a Janus thermal emitter to act as a selective emitter (SE) on the top and as a broadband emitter (BE) on the bottom. The design efficiently drew heat from the inner space and surface, while the top emitted heat to space without disturbing the ambient radiation.
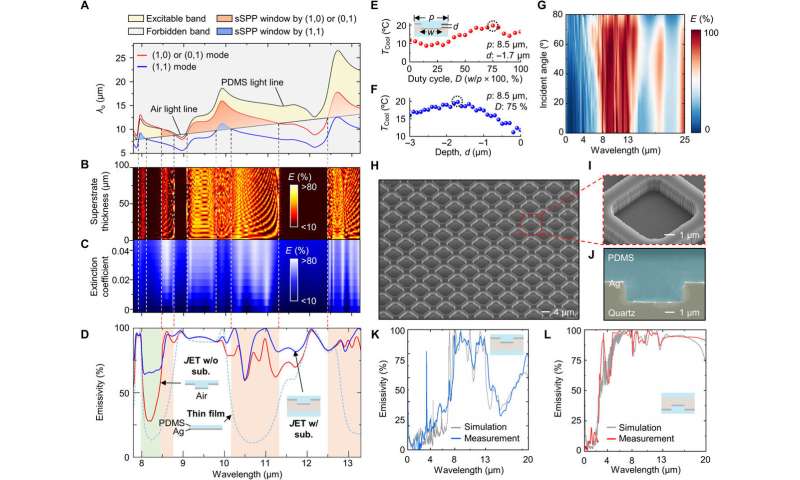
JET for cooling enclosed spaces and sSPP resonance for near-ideal selective emitters
The scientists first designed a polymer-based selective emitter (SE) incorporating spoof surface plasmon polariton (sSPP) to achieve near-ideal selectivity. Then they theoretically and experimentally showed the cooling performances of the Janus emitter (JET) on both sides, much like state-of-the-art radiative coolers. The JET functioned as an effective heat channel to absorb broadband thermal radiation from the interior and bottom, while using the top-side to radiate heat as infrared (IR) waves to outer-space, much like a cold sink. The sample contained a polydimethylsiloxane (PDMS) layer, a 100 nm thick silver layer and a micropatterned quartz layer coated with 10 µm-thick PDMS on the bottom. The JET minimized the disturbance from solar energy and ambient radiation, where the bottom-side broadly adsorbed inner thermal radiation. The team calculated the cooling powers and cooling temperatures for the selective emitter (SE) and broadband emitter (BE) during the study.
Heo et al. analyzed the effects of spoof surface plasmon polariton (sSPP) resonances on JET emissivity and the simulation showed strong resonant absorption peaks excited between the two sSPP modes, due to the Fabry–Pérot cavity resonance of the setup. The JET showed angle-robust emissivity near the atmospheric window. Using scanning electron microscopy (SEM) images they observed the micropatterned quartz with or without PDMS coating. The measured and simulated emissivity spectra indicated near-ideal features in both selective emitters (SE) and broadband emitters (BE) of the fabricated JET system.
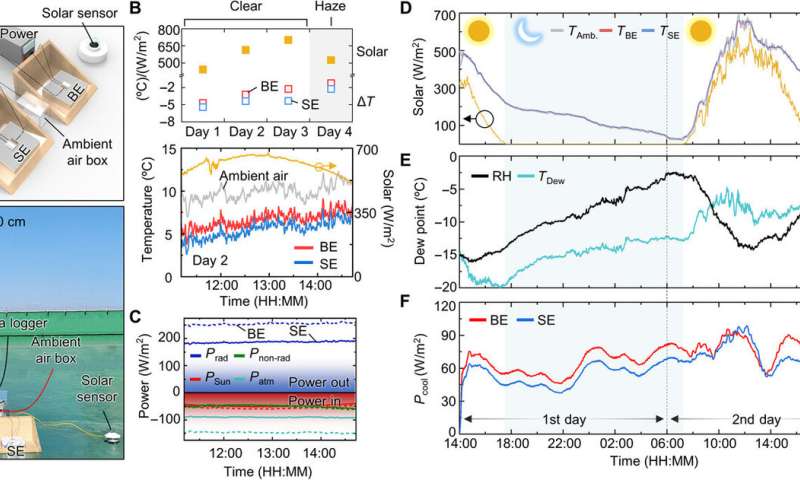
Proof-of-concept: Surface cooling performance of JET in SE and BE
To examine the cooling power and cooling temperature of both selective emitters and broadband emitters (SE and BE) in the device, the scientists accessed an outdoor rooftop at the Gwangju Institute of Science (GIST). The team prevented self-heating of the ambient air sensor by using an ambient air box to shade the solar spectrum and provided continuous air flow to the setup. They tested the temperature sensors for reliability and did not use a convection shield due to imperfect transmittance. The results showed sub-ambient cooling under different weather conditions, where haze and humidity inhibited heat transfer to the atmosphere. Heo et al. classified the steady-state energy balance equation into four power terms, including the (1) power emitted by the sample, (2) power absorbed by atmospheric emission, (3) absorbed power of solar irradiation and (4) non-irradiation heat transfer, which included conduction and convection. The SE was more effective during sub-ambient cooling compared to BE. The team measured the cooling power alongside climate conditions during the experiments.
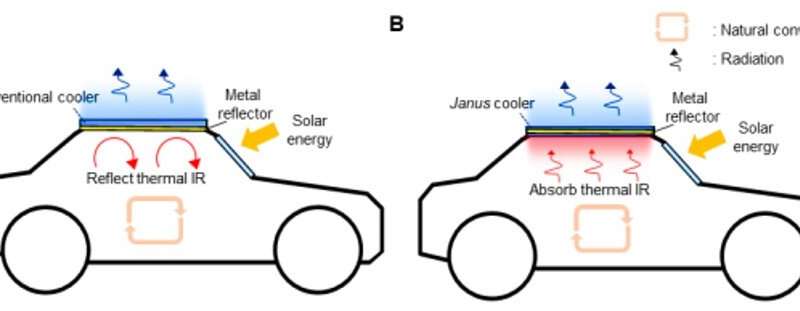
Cooling capability of JET in an enclosed space
Although heat transfer mainly occurs via convection in open areas, the mechanism can differ in an enclosed space with a heating source. For example, an automobile parked under the sun can heat from 60 degrees up to 80 degrees Celsius, although the ambient temperature is only 21 degrees Celsius, causing hyperthermia in occupant children. During Janus thermal radiation, the JET (Janus emitters) can function as a heat channel to draw heat from the enclosure and significantly alter temperature distribution in the inner region. The JET is highly efficient in dropping temperature from the shielded area via broadband absorption and allowing selective thermal emission through the atmospheric window.
The team developed an experimental model using aluminum metal and black leather to mimic a stationary vehicle interior and floor, parked under the sun. They performed the experiment on a rooftop and noted the exceptional cooling performance of JET in the enclosed space repetitively on four different days under different weather conditions. Based on the outcomes, the team proposed replacing the material used here with other polymers for a variety of optimized benefits, including an enhanced overall cooling capacity by minimizing solar power and increasing thermal radiation. The surface properties of the JET also provided water-proofing and self-cleaning effects.
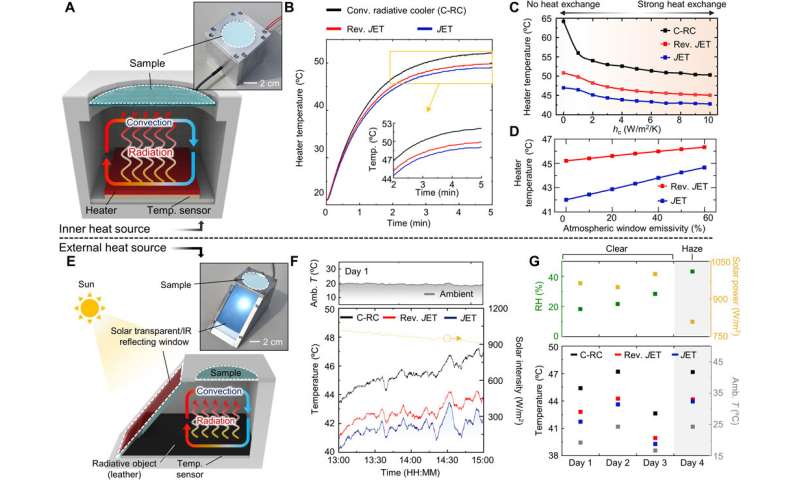
In this way, Se-Yeon Heo and colleagues showed how Janus emitters provided a passive strategy for selective emission to outer space, alongside broadband absorption on the opposing side of the enclosure. To accomplish this, they developed an almost-ideal selective emitter (SE) with spoof surface plasmon polariton (sSPP) within a PDMS polymer clad on a silver-coated micropatterned quartz frame for the experiments. They examined the capability of JET to cool enclosures, where it drew heat away compared to other materials. Using the bidirectional emission characteristics of Janus emitters, the team lowered the temperature of a radiative object in an enclosure which simulated a stationary automobile environment. The superior capability to passively cool both top and bottom surfaces as well as enclosed spaces can allow the development of advanced designs to minimize the greenhouse effect in enclosed spaces such as stationary automobiles.
More information: 1. Heo S. et al. A Janus emitter for passive heat release from enclosures, Science Advances, 10.1126/sciadv.abb1906
2. Bhatia B. et al. Passive directional sub-ambient daytime radiative cooling, Nature Communications, doi.org/10.1038/s41467-018-07293-9
3. Smith G. & Gentle A. Radiative cooling: Energy savings from the sky, Nature Energy, doi.org/10.1038/nenergy.2017.142
Journal information: Science Advances , Nature Communications , Nature Energy
© 2020 Science X Network